Concurrent Development and Assessment of Emerging Technologies:
Case of Carbon Nanotubes
Today, advanced innovative researches have enabled a wide possibility to revolutionize technologies. However, the conventional focus of scientists on technical performances such as material characteristics often neglects the environmental consequences of the changes, which may lead to a wastage of resources. These can be easily prevented via decision-making based on early-stage life cycle assessment (LCA). Here, we share our experience in pioneering such collaborative work. We present a framework for concurrent development and (environmental) assessment of emerging technologies (Figure 1). We then apply it to the case of carbon nanotubes (CNT). We highlight that breaking the thinking in disciplinary silos is critical to sustainable technology; LCA plays the role in bridging scientific knowledge to our climate-emergency society.
The case of CNT. CNT is an emerging material with promising applications due to its superior properties. Mass production of CNT is most feasible with catalytic chemical vapor deposition (CVD). To synthesize high-quality single-wall to few-wall CNT, catalyst nano particles must first be deposited on substrates. Then, using a high-temperature CVD reactor, hydrocarbons are decomposed into CNT. The processes are intensive in material and energy. Yet, the consequential impact on the environment is rarely studied because of uncertainties in syntheses. Leveraging the experience in lab syntheses, this study aimed to evaluate the prospective environmental impact of CNT synthesized via three selected catalytic CVD methods. First, the CO2-assisted growth on flat substrates (on-substrate CVD) [1], represented the established millimeter long CNT growth. Second, the fluidized-bed growth on spherical beads (fluidized-bed CVD) with sputtered catalysts [2], contrasted the effect of 3D CNT growth to previous 2D growth. Third, the fluidized-bed CVD with chemical vapor deposited catalysts [3], showed a repeatable single-reactor production. We quantified the global warming potential impact of 1 g CNT production with LCA. The results for the three syntheses were 28.55, 9.94, and 2.10 kg-CO2equiv/g-CNT, accordingly (Figure 2). By comparing the three syntheses, we showed that the strikingly high impacts were mitigated through increasing CNT yield with fluidized bed and replacing the energy-intensive sputtering process. A scenario to substitute the remaining hotspot, argon (purging gas), with nitrogen could reduce the impact to 0.48 kg-CO2equiv/g-CNT. When considering scale-up from lab-scale (reactor diameter of 22 mm, productivity of 5 g per day) to industrial scale (diameter >30 times, productivity >3 to 104 times), the CNT production would be comparable to industrialized carbon fiber production about 0.02 kg-CO2equiv/g-CF.
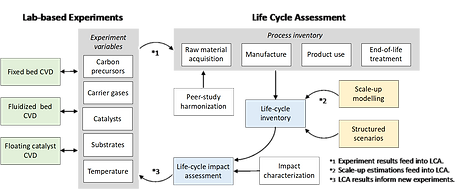

Figure 1: Proposed framework for concurrent development and assessment of emerging technologies; using carbon nanotubes synthesis as an example.
Figure 2: Summary of life cycle GHG emissions of 1 g CNT with low-carbon strategy.
​References:
-
Sato T, Sugime H, Noda S. CO2-assisted growth of millimeter-tall single-wall carbon nanotube arrays and its advantage against H2O for large-scale and uniform synthesis. Carbon. 2018 Sep 1;136:143-9.
-
Kim DY, Sugime H, Hasegawa K, Osawa T, Noda S. Fluidized-bed synthesis of sub-millimeter-long single walled carbon nanotube arrays. Carbon. 2012 Apr 1;50(4):1538-45.
-
Kim DY, Sugime H, Hasegawa K, Osawa T, Noda S. Sub-millimeter-long carbon nanotubes repeatedly grown on and separated from ceramic beads in a single fluidized bed reactor. Carbon. 2011 May 1;49(6):1972-9.
Dr. Heng Yi Teah is an Assistant Professor at Waseda Research Institute for Science and Engineering. He is affiliated to Department of Applied Chemistry in Waseda University. He was previously trained in Taiwan during 2009-2014—BSc + MSc in Environment Engineering at National Cheng Kung University. He later received his PhD in Sustainability Science at the University of Tokyo in 2017. His primary research interests are life cycle assessment (LCA) and sustainability assessment; particularly on inter- to transdisciplinary collaborative research. Currently, he is working on technology assessment of emerging technologies such as carbon nanotubes enabled devices. He works closely with basic sciences and applied sciences research groups to bridge the gap of disciplinary knowledge for a sustainable future. Although he is an early-career scientist, he has been awarded several competitive grants related to the themes above, including JSPS DC/PD Fellowship, Kakenhi Start-up (Japan), and Proactive MAGIC (MOST, Taiwan).
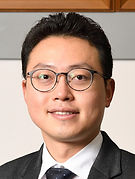
Heng Yi Teah, PhD
Assistant Professor
Waseda Research Institute for Science and Engineering
Waseda University
Tokyo, Japan
Email: teah[at]aoni.waseda.jp
Biodiesel Production from Waste Cooking Oils by Using the Ultrasound Technique; Synthesis of NaA and NaY Zeolites from Kaolin Natural Mineral and Use as Absorbent for Heavy Metal Removal
Petro-diesel fuel emissions have serious adverse environmental effects. Biodiesel is renewable and easily biodegradable, and helps to reduce a country’s reliance on crude oil imports and supports agriculture by providing a new market for domestic crops. The objective of our research was to produce biodiesel satisfying the biodiesel-fuel standards with low energy consumption and material savings. Transesterification reaction of waste cooking oils with methanol in the presence of base catalyst was accelerated by an ultrasonic reactor at low frequency 20 kHz with input capacity of 1kW, and was carried out by two steps process. For the first step, the molar ratio of methanol to oil of 3:1 and amount of KOH 0.75 wt.% (KOH to oil) were used, and the yield of fatty acid methyl esters (FAME) was about 90%. For the second step the yield of FAME can be obtained more than 99% with molar ratio of methanol to initial oil of 1:1 and amount of KOH 0.25 wt.%. It is surprising that the FAME yield was extremely high even short retention time of reaction mixture in the reactor (less than 80 sc. for both steps) at room temperature. The quality of final product cleared the standard JIS K 2390 for biodiesel fuel.
Zeolite, a microporous crystalline material, has been using as catalyst, absorbent and ion exchange material. Here we report the synthesis of NaA (LTA) and NaY (FAU) zeolites from kaolin natural mineral and use as absorbent for heavy metal removal. Batch experiment was carried out to study the adsorption of metal ions including Pb2+, Cd2+, Zn2+, Cu2+, Sr2+, Cs+, and the adsorption parameters such as contact time, initial metal concentration were investigated. The results showed that adsorption capacity of zeolite NaA is much higher than that of zeolite NaY. The removal efficiency of these zeolites at low metal ion concentration (50 ppm) of Pb2+, Cd2+ was above 99%. At high concentration (500 ppm), the maximum adsorption capacity for Pb2+ is 533.1 mg/g and 302.1 mg/g for zeolite NaA and NaY, respectively. Materials showed stable adsorption, in-reversible process, and suitable absorbent for heavy metal capture and storage.
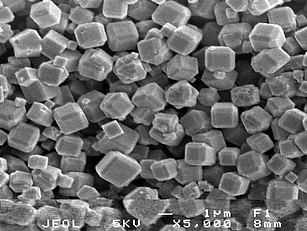
NaA

NaY
Dr. Le Tu Thanh received his PhD in chemical engineering from the Graduated School of Engineering, Osaka Prefecture University, Japan. Currently, he is a lecturer, and head of Environmental Science Department, Faculty of Environment, University of Science, Vietnam National University – Ho Chi Minh City. Dr. Thanh’s research interest is development of the innovation methods for producing high quality of biodiesel fuel from inexpensive materials such as waste cooking oils and animal fats. Recently, his new research interest is synthesis of zeolites from kaolin natural material and fly ash from power plants, and the utilization of zeolites in removing heavy metals from wastewater and the use of zeolites in gas mixtures separation.

Le Tu Thanh, Dr. Eng.
Head, Department of Environmental Science
Faculty of Environment
University of Science, VNU-HCM
Hochiminh City, Vietnam
Email: letuthanh[at]hcmus.edu.vn
Hydrothermal Reaction/Extraction/Leaching for Circular Economy
Circular economy is proposed by EU [1] it is used again to create further value and it is described as: “the value of products and materials is maintained for as long as possible. Waste and resource use are minimized, and when a product reaches the end of its life, it is used again to create further value. This can bring major economic benefits, contributing to innovation, growth and job creation”. Here, “resource” is fossil and mining materials and its use should be minimized. Instead, in circular economy it is said that “the end-of-life materials must be used again to create further value”. In the presentation, Resources are now defined as not only conventional (fossil fuels such as petroleum) but also unused (biomass and waste). That means, bulk and huge amount of materials such as petroleum, biomass and waste (by-products) could be resources. From this point of view, resources are always complicated polymeric mixtures. Thus, for the circular economy, resources are required to be valorized. To do so, separation (decomposition, fragmentation, solubilization, extraction, leaching etc) is necessary for effective and industrial utilization. For petroleum, hydrocarbons can be separated with distillation because these can be vaporized depending on their physical properties, that is, boiling points. But, for unused resources such as biomass and wastes, the separation of these resources into each component is quite difficult because the components in the unused resources are not easily vaporized and the optional or brand-new technology for the separation should be developed.
Hydrothermal water is an environmental benign solvent and solubilization power and reactivity are quite high for the various kinds of components not only for organics but also inorganics. Thus, for the separation of unconventional unused resources, hydrothermal process has been hardly studied. In this presentation, some research topics concerning hydrothermal technologies such as reactions [2], extraction [3], leaching (lithium ion battery cathode materials is leached with organic acid in hydrothermal water) will be introduced.
​
References:
-
https://ec.europa.eu/growth/industry/sustainability/circular-economy_en
-
Masaru Watanabe, Masayoshi Wagatsuma, Keisuke Suzuki, Takuma Kato, Yasuto Goto, Yukihiro Kanaguri and Yuya Hiraga, Resource Upgrading in Advanced Supercritical Fluid (Supercritical Fluid with Catalyst and Cosolvent): Liquid Fuels from Biomass in Sub and Supercritical Water and Carbohydrate Up-Conversion in Ionic Liquid and Supercritical Fluids Mixtures, Advanced Supercritical Fluids Technologies, Intech, DOI: 10.5772/intechopen.89793.
-
M. Watanabe, Y. Kanaguri, R. L. Smith: Hydrothermal separation of lignin from bark of Japanese cedar. J. Supercrit. Fluids, 2018:133: 696-703.
Qualifications:
-
Ph D thesis in Chemical Engineering (Tohoku University) (1999)
-
Industrial chemistry M Sc (Meiji University) (1995)
​
Professional Experience:
-
[2000-2006] Assistant Professor in Research Center of Supercritical Fluid Technology, Department of Chemical Engineering, Tohoku University
-
[March to August 2005] Guest researcher in Forschungszentrum Karlsruhe, Germany
-
[2006-2018] Associate Professor in Research Center of Supercritical Fluid Technology, Department of Chemical Engineering, Tohoku University
-
[May 2018] Full Professor in Research Center of Supercritical Fluid Technology, Department of Chemical Engineering, Tohoku University
​
Research Topics:
-
Resource conversion in sub and supercritical water
-
Hydrothermal catalytic reaction of biomass
-
Recycle and valorization of waste materials with hydrothermal and sub-/supercritical water technology
​
Achievements:
-
Co-author of 104 articles in peer-reviewed international scientific journals
-
Co-author of 5 Patents, 3 Book chapters
-
Supervision or co-supervision of Ph D thesis: 6 completed
-
Bice char of division of supercritical fluid in Japan Society of Chemical Engineers
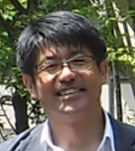
Masaru Watanabe, Dr. (Eng)
Professor
Research Center of Supercritical Fluid Technology & Department of Chemical Engineering, Graduate School of Engineering
Tohoku University
Sendai, Japan
Email: masaru.watanabe.e2[at]tohoku.ac.jp
Life Cycle Assessment 2.0: From Product Management Tool to Systems Design Tool
In the past, the industry has been producing their products in such a way to maximize profits while considering environmental aspects as constraints that must be satisfied. Nowadays, this type of problem formulation needs reconsideration; sometimes, environmental aspects are not merely constraints. For example, some processes are introduced, aiming at increasing the renewability of the resources consumed or at lowering carbon emission to mitigate climate change. In designing an innovative system to lead the societies towards a sustainable vision, consideration of environmental aspects, as well as economic and social aspects, are necessary. Life Cycle Assessment (LCA) is increasingly used to serve in such a context, introducing a system-wide environmental perspective in technology and technical systems assessment.
LCA has been developed as an environmental systems analysis tool specialized in product system management. It aims to understand the system-wide environmental intervention of human activities. Conventional LCA (hereafter referred to as LCA 1.0) is characterized by its product-centered view on economic activities. The scope of the study encompasses the “cradle-to-grave (mining, transportation, manufacturing, retail, use, recycle, end of life treatment, ...)” of a target product. In LCA 1.0, it is essential to clarify what the “functional unit” is. The functional unit can be a tangible product (ex. a personal vehicle), or a service (ex. 1 km transportation of 1 person by a personal vehicle). There have been numerous studies conducted using LCA 1.0 because it takes into account both direct and indirect environmental burdens induced by the functional unit.
While providing a simple view of complex industrial systems, there are also some drawbacks to the use of a product-centered view on a system. One of the important ones among several is that it tends to over-emphasize the efficiency aspect when it is applied to the evaluation of the technologies used in the product life cycle. For example, there has been a “3R (reduce, reuse, recycle) policy” for recycling in Japan. Under LCA 1.0 approach, various recycling routes are ranked by its efficiency in reducing environmental burdens, in many case greenhouse gases emission in Japan. The metric used here is, for example, kg-CO2eq. / kg-product. However, in Japan, we have learned in the past 2 decades that this approach overemphasizes the efficiency of recycling, and the stakeholders tend to interpret the results in such a way to overlook the importance of comprehensiveness in the recycling system.
Here, I claim that for systems design purpose, it is necessary that the LCA must depart from its product-centered approach (creating solutions that delivers the equivalent functional unit), and evolve into a systems-oriented approach (creating solutions that satisfies the equivalent set of constraints), while maintaining its comprehensive perspective on the associated environmental impacts. As a new approach, I propose an enhanced framework of LCA tailored for systems design (LCA 2.0). A system can produce and utilize the different amount of products (i.e., the system alternatives do not have to be consistent on the functional units), while satisfying design requirements on the system, such as economic productivity, environmental burdens, available resources, etc. During design activity, these constraints will be reviewed by multiple stakeholders for its comprehensiveness, sensitivity, and balances among various indicators. I will introduce how conventional LCA has been dealing with such assessment needs and share the new strategy taken by the proposed method.
The development of chemical and environmental technologies is one of the essential keys to realize a sustainability shift in society. To effectively optimize the technologies for a system that would utilize the technology, introducing a systems perspective is necessary. In this lecture, I would also try to communicate the audience the joy and excitement of chemical/environmental systems study, as well as the benefit of technology development researchers to work in partnership with such a group.
Dr. Fukushima is an Associate Professor of Chemical Systems Engineering at the Department of Chemical Engineering, Tohoku University, Japan. He attended The University of Tokyo since 1993 and earned his doctorate in 2002. After serving as a research associate at the University of Tokyo for two years, Dr. Fukushima moved to National Cheng Kung University, Taiwan, in 2004. He taught as Assistant/Associate Professor in the Department of Environmental Engineering, till he moved to the current position in Tohoku University Japan in 2014. He is interested in the development of simulation and assessment methods and tools for innovative systems design, aiming at the construction of sustainable societies utilizing locally available resources that are unexploited at the moment.
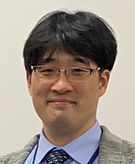
Yasuhiro Fukushima, PhD
Associate Professor of Chemical Systems Engineering
Department of Chemical Engineering
Graduate School of Engineering
Tohoku University